Introduction to Hydrogen Embrittlement in Steel Pipes
Overview of hydrogen embrittlement phenomenon
Hydrogen embrittlement is a complex process by which the presence of hydrogen can drastically reduce the ductility and load-bearing capacity of steels and other metals, causing cracking or catastrophic brittle failures at stresses below the yield strength of the material. The small hydrogen atoms can easily penetrate and diffuse through the metal’s crystal lattice, migrating to microstructural defects where stresses concentrate. The accumulation of hydrogen at these locations then weakens or damages the cohesive bonds between the metal atoms, leading to loss of ductility and crack initiation and propagation.
Although hydrogen embrittlement has been recognized for over 140 years, the specific mechanisms driving crack initiation and growth are still not completely understood. However, it is clear that many common steels and metal alloys are susceptible to hydrogen embrittlement damage, especially under sustained loading or cyclic stresses. Preventing hydrogen ingress and limiting internal hydrogen concentrations are critical for ensuring the safe performance and reliability of high-strength steels used in demanding applications.
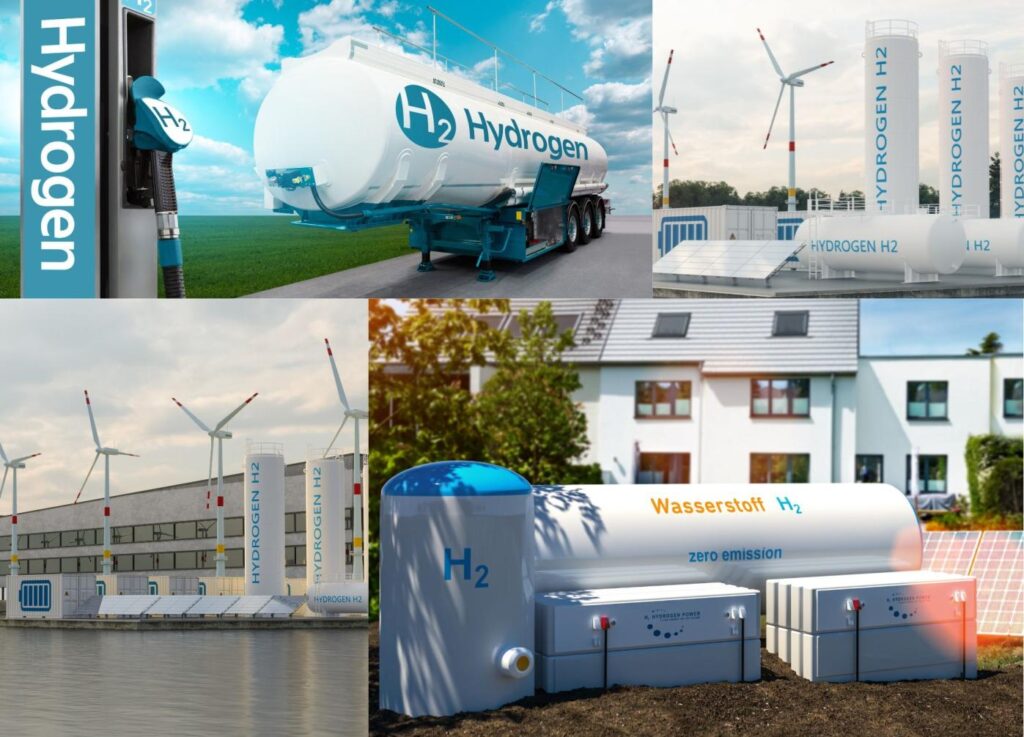
Susceptibility of high-strength pipeline steels
Pipeline steels transporting gaseous hydrogen are exposed directly to atomic hydrogen and at risk for hydrogen environment assisted cracking. Newer grades of high-strength pipeline steels such as API X80 and X100, with minimum specified yield strengths up to 690 MPa and 760 MPa respectively, are more prone to hydrogen embrittlement damage compared to lower strength pipeline steels.
Factors that increase susceptibility to hydrogen embrittlement in pipeline steels:
- High strength levels – Higher strength steels have lower fracture toughness that is more easily degraded by hydrogen effects.
- Microstructure – Bainitic, tempered martensitic, and tempered lower bainite microstructures are more resistant than ferrite-pearlite.
- Strength mismatches – Mismatches between pipe body, girth weld, and heat-affected zone strengths can create localized stress concentrations for hydrogen damage.
- Residual stresses – Welding processes introduce residual stresses that compound with applied stresses.
- Cyclic stresses – Fluctuating pressures and demands create fatigue conditions that accelerate hydrogen cracking.
- Surface defects – Cracks, dents, gouges, and other surface defects concentrate stresses.
Impact on mechanical properties and integrity
The effects of hydrogen on the mechanical performance of steel pipes include:
- Lower ductility and fracture toughness – Hydrogen reduces the strain energy required for crack initiation and growth, decreasing fracture resistance.
- Loss of tensile strength – Hydrogen accumulation at microvoids and defects allows cracks to propagate at reduced stresses.
- Accelerated fatigue crack growth – Cyclic stresses drive hydrogen diffusion to crack tips, increasing growth rates.
- Increased brittleness and hardness – Hydrogen promotes yield point phenomena that increase localized hardness.
- Crack coalescence – Micro-cracks initiated at defects link together as main cracks.
- Localized plastic instability – Hydrogen enhances dislocation mobility, promoting strain localization.
At sufficient levels, these hydrogen-induced effects can lead to unexpected brittle failures and catastrophic ruptures of steel pipes and vessels under stresses normally tolerated. Carefully managing hydrogen exposure, controlling microstructure, and applying protective coatings are key to mitigating embrittlement risks.
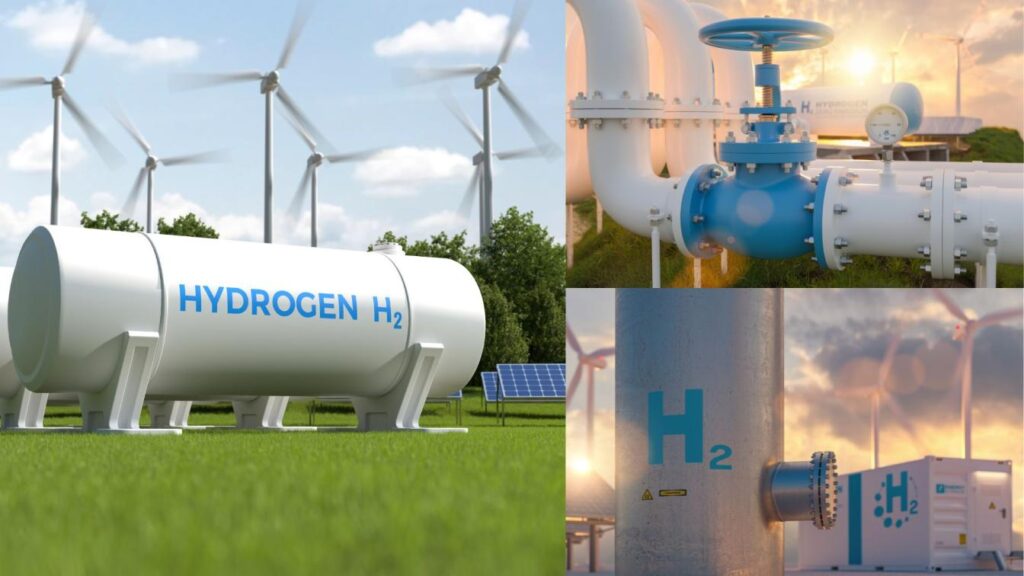
Mechanisms of Hydrogen Damage in Steel Pipes
Hydrogen environment assisted cracking
Also known as hydrogen stress cracking, this occurs when atomic hydrogen is generated through corrosion reactions with the steel’s surface. Hydrogen atoms diffuse and accumulate at regions of high triaxial tensile stress ahead of cracks and defects, weakening interatomic bonding and initiating microvoids that grow and coalesce. These micro-cracks then propagate rapidly under sustained tensile stresses, overlapping in brittle transgranular or intergranular fracture.
Hydrogen entry and diffusion
Sources of atomic hydrogen exposure include:
- High-pressure gaseous hydrogen environments in gas pipelines.
- Aqueous corrosion generating atomic hydrogen that penetrates the steel surface.
- Stray currents from cathodic protection accelerating the hydrogen evolution reaction.
- Welding processes that introduce hydrogen from moisture and organic contaminants.
Hydrogen permeation into pipeline steels occurs through:
- Lattice diffusion – Interstitial migration through the iron lattice matrix.
- Grain boundary diffusion – Faster diffusion along microstructural discontinuities.
- Pipe surfaces – Entry facilitated by defects, strain concentrations, and corrosion damage.
Hydrogen pressure effects
- Pressures above ~100 bar in gas transmission pipelines increase the risk of hydrogen environment assisted cracking.
- Cyclic pressures from fluctuating gas flow create fatigue conditions accelerating damage.
- When pressure is rapidly reduced, the resulting concentration gradient causes high internal hydrogen diffusion rates.
Hydrogen-microstructure interactions
- Microstructures and phases affect hydrogen solubility, diffusivity, and trapping.
- Dislocations, carbides, inclusions, and other defects provide deep trapping sites.
- Mismatches in hardness and hydrogen solubility between weld, HAZ, and base metal impact local susceptibility.
Hydrogen embrittlement vs hydrogen stress cracking
While similar in effects, some distinctions exist:
- Hydrogen embrittlement is driven by pre-existing internal hydrogen, while hydrogen stress cracking requires sustained hydrogen ingress from the environment.
- Hydrogen embrittlement involves distributed microstructural damage ahead of cracks; hydrogen stress cracking is more localized crack tip damage.
- For pipeline steels, the mechanisms usually act synergistically rather than distinctly.
Hydrogen blistering and bulging in storage vessels
Accumulation of atomic hydrogen during aqueous corrosion or welding processes can generate high internal hydrogen pressures. This leads to bulging or blister cracking failures in tanks and other closed vessels.
So in summary, even at relatively low levels hydrogen can severely degrade the structural integrity and fracture resistance of steel pipes and vessels under pressure and stress. Understanding embrittlement mechanisms is key to failure prevention and ensuring safety.
Gas Blending is Not a Solution for Hydrogen Transportation via Steel Pipelines
There is growing interest in blending hydrogen into natural gas pipelines as a way to utilize existing infrastructure for hydrogen delivery. However, significant technical and safety challenges exist with gas blending that prevent it from being a viable solution for steel pipeline hydrogen transportation.
Blending Impacts Steel Pipe Integrity through Hydrogen Embrittlement
One of the biggest risks with hydrogen-natural gas blends is hydrogen embrittlement of the steel pipelines. Hydrogen causes the steel to become brittle and prone to cracking failures through several mechanisms:
- Hydrogen atoms diffusing into the steel reduce ductility and fracture toughness. Even small concentrations of a few ppm can begin degrading the steel’s properties.
- Atomic hydrogen accumulates at microstructural defects, initiating cracks that propagate rapidly under cyclic stresses.
- Hardness and strength are increased locally, while global ductility is reduced through hydrogen-enhanced dislocation mobility and strain localization.
Factors impacting hydrogen embrittlement risks with blending:
- Higher blend concentrations accelerate damage rates and reduce the threshold for cracking.
- Greater pipeline pressures drive more hydrogen into the steel.
- Cyclic pressures from fluctuating flow create fatigue conditions.
- High-strength steels are more susceptible to hydrogen cracking.
Hydrogen embrittlement caused by blending can ultimately lead to sudden pipeline failures, leaks, and ruptures.
Hydrogen Leakage and Ignition Hazards Increase with Blending
In addition to steel pipe embrittlement, hydrogen blending creates safety risks due to leakage and ignition hazards:
- Blends containing over 20% hydrogen have greater leakage potential through seals, welds, and plastic components in the gas infrastructure.
- Leaked hydrogen has a very wide flammability range and can auto-ignite in air. It burns rapidly with an invisible flame.
- Hydrogen concentrations from leaks disperse and dilute quickly to create an even larger flammable envelope.
These risks mean that even small hydrogen leaks from blending have high consequences in terms of fire and explosion potential. Leak prevention and mitigation measures would require extensive upgrades.
Gas Transmission and Distribution Systems Are Not Designed for Hydrogen Service
Natural gas infrastructure has not been designed, constructed, or maintained for hydrogen service:
- Pipeline grades, welding methods, and maintenance practices are optimized for natural gas – not hydrogen – transportation. Conversion requires significant capital investment.
- Gas compressor seals, valves, meters, and regulator components are subject to hydrogen embrittlement failures and leakage.
- Pipeline corrosion and crack growth rates can be accelerated by hydrogen exposure.
Customer End-Use Equipment Poses Additional Blending Risks
Hydrogen blending affects downstream gas utilization equipment:
- Most appliances are only designed for natural gas fuel. Burner modifications and materials upgrades would be needed to handle hydrogen fuel.
- Leakage potential increases in homes from elastomer seals and connections.
- Operation outside of design parameters increases risks of inefficient combustion or equipment damage.
Extensive testing, retrofitting, or replacement would be necessary to safely handle hydrogen blends.
Other Operational and Economic Impacts of Hydrogen Blending
Blending hydrogen into natural gas also introduces other challenges:
- Reduced heating value from hydrogen dilution decreases energy delivery per volume of blended gas.
- Variations in blend ratios require precise monitoring to control combustor performance.
- Increased processing is needed to maintain pipeline gas quality specifications under blending.
- Embrittlement and leakage risks create public safety concerns that challenge regulatory approval.
When considering the above impacts on safety, operations, and economics, it becomes clear that hydrogen blending provides minimal benefits compared to the substantial technical and cost barriers for implementation. Construction of dedicated hydrogen pipelines and distribution systems engineered specifically for hydrogen gas is a more prudent investment path.
Key Takeaways – Why Gas Blending is Not the Solution
- Hydrogen embrittlement – Blending introduces embrittlement risks that compromise steel pipe integrity at higher hydrogen levels.
- Ignition hazards – Leakage potential increases with more hydrogen, creating flammability and explosion risks.
- Infrastructure compatibility – Existing natural gas systems were not designed for any significant hydrogen exposure.
- End-use equipment – Components pose ignition risks; efficiency losses likely without modification.
- Operational challenges – Heating value, quality variability, and flow management issues.
- Limited benefit for costs – Blending provides minimal advantage versus dedicated hydrogen pipelines.
Overall, blending hydrogen into the natural gas network is too problematic from an engineering standpoint and provides minimal payoff. Purpose-built hydrogen infrastructure is required for large-scale hydrogen transportation.
FAQ: Hydrogen Embrittlement in Steel Pipelines
- What is Hydrogen Embrittlement?
Hydrogen embrittlement, also known as hydrogen-induced cracking or hydrogen-assisted cracking, is a phenomenon in which steel pipelines become brittle and prone to fracture due to the presence of hydrogen. - How does Hydrogen Enter Steel Pipelines?
Hydrogen can enter steel pipelines through various sources, including corrosion, exposure to hydrogen gas, and hydrogen pickup during manufacturing and processing. - What Are the Effects of Hydrogen Exposure on Pipeline Steels?
The exposure of pipeline steels to hydrogen can lead to various detrimental effects, including reduced ductility, increased susceptibility to cracking, and overall material degradation. - What is Hydrogen Embrittlement Resistance?
Hydrogen embrittlement resistance refers to the ability of steel pipelines to withstand the damaging effects of hydrogen exposure without experiencing a loss of structural integrity. - How Does Hydrogen Permeation Affect Steel Pipes?
Hydrogen permeation refers to the diffusion of hydrogen through the steel pipe’s material. This process can lead to the accumulation of hydrogen, which can cause embrittlement over time. - What Are the Mechanisms Behind Hydrogen-Induced Cracking?
Hydrogen-induced cracking mechanisms involve the interaction of hydrogen with the steel’s microstructure, leading to various cracking phenomena. These mechanisms are crucial to understanding and preventing hydrogen-related failures. - How Can Hydrogen-Induced Damage be Mitigated?
Mitigating hydrogen-induced damage involves using hydrogen-resistant alloys, coatings, and steel grades. It also includes proper design and maintenance practices to minimize hydrogen exposure. - What Testing Methods are Used to Assess Hydrogen Damage?
Hydrogen damage testing methods, such as permeation, diffusion, and microstructure interactions testing, help evaluate the susceptibility of steel pipelines to hydrogen embrittlement. - What Are the Common Hydrogen-Resistant Materials and Coatings?
Common hydrogen-resistant materials and coatings include alloys specifically designed to resist hydrogen embrittlement, as well as protective coatings that act as barriers to prevent hydrogen penetration.
https://www.sciencedirect.com/science/article/pii/S0921509322016422
https://www.sciencedirect.com/science/article/abs/pii/S0360319920316980